
Poly(p-phenylene) (PPP) is made of repeating p-phenylene units, which act as the precursor to a conducting polymer of the rigid-rod polymer family. The synthesis of PPP has proven challenging, but has been accomplished through excess polycondensation with the Suzuki coupling method.

Dendrimers are highly ordered, branched polymeric molecules. Synonymous terms for dendrimer include arborols and cascade molecules. Typically, dendrimers are symmetric about the core, and often adopt a spherical three-dimensional morphology. The word dendron is also encountered frequently. A dendron usually contains a single chemically addressable group called the focal point or core. The difference between dendrons and dendrimers is illustrated in the top figure, but the terms are typically encountered interchangeably.

In chemistry, a supramolecular assembly is a complex of molecules held together by noncovalent bonds. While a supramolecular assembly can be simply composed of two molecules, or a defined number of stoichiometrically interacting molecules within a quaternary complex, it is more often used to denote larger complexes composed of indefinite numbers of molecules that form sphere-, rod-, or sheet-like species. Colloids, liquid crystals, biomolecular condensates, micelles, liposomes and biological membranes are examples of supramolecular assemblies, and their realm of study is known as supramolecular chemistry. The dimensions of supramolecular assemblies can range from nanometers to micrometers. Thus they allow access to nanoscale objects using a bottom-up approach in far fewer steps than a single molecule of similar dimensions.

Reversible addition−fragmentation chain-transfer or RAFT polymerization is one of several kinds of reversible-deactivation radical polymerization. It makes use of a chain-transfer agent (CTA) in the form of a thiocarbonylthio compound to afford control over the generated molecular weight and polydispersity during a free-radical polymerization. Discovered at the Commonwealth Scientific and Industrial Research Organisation (CSIRO) of Australia in 1998, RAFT polymerization is one of several living or controlled radical polymerization techniques, others being atom transfer radical polymerization (ATRP) and nitroxide-mediated polymerization (NMP), etc. RAFT polymerization uses thiocarbonylthio compounds, such as dithioesters, thiocarbamates, and xanthates, to mediate the polymerization via a reversible chain-transfer process. As with other controlled radical polymerization techniques, RAFT polymerizations can be performed under conditions that favor low dispersity and a pre-chosen molecular weight. RAFT polymerization can be used to design polymers of complex architectures, such as linear block copolymers, comb-like, star, brush polymers, dendrimers and cross-linked networks.
Barrelene is a bicyclic organic compound with chemical formula C8H8 and systematic name bicyclo[2.2.2]octa-2,5,7-triene. First synthesized and described by Howard Zimmerman in 1960, the name derives from the resemblance to a barrel, with the staves being three ethylene units attached to two methine groups. It is the formal Diels–Alder adduct of benzene and acetylene. Due to its unusual molecular geometry, the compound is of considerable interest to theoretical chemists.

The enzyme horseradish peroxidase (HRP), found in the roots of horseradish, is used extensively in biochemistry applications. It is a metalloenzyme with many isoforms, of which the most studied type is C. It catalyzes the oxidation of various organic substrates by hydrogen peroxide.

Polystannanes are organotin compounds with the formula (R2Sn)n. These polymers have been of intermittent academic interest; they are unusual because heavy elements comprise the backbone. Structurally related but better characterized (and more useful) are the polysilanes (R2Si)n.
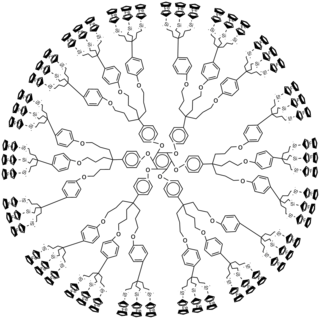
Ferrocene-containing dendrimers are dendrimers that contain ferrocene substituents. Some ferrocene-containing dendrimers feature ferrocene cores and others do not. All feature with peripheral ferrocene groups.
Catalytic chain transfer (CCT) is a process that can be incorporated into radical polymerization to obtain greater control over the resulting products.

Established in 2005, Polymer Factory concentrates on developing well defined dendrimers and dendron based on 2,2-bis(methylol)propionic acid, where the company has the exclusive right to the production, marketing, and sales of such materials. The company also provides tailor-made hyperbranched polymers. Polymer Factory's research lab is located in Stockholm, Sweden.
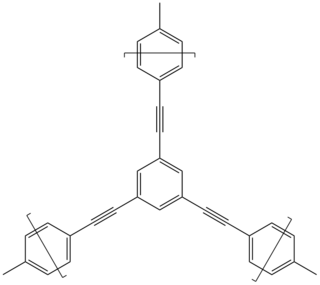
Conjugated microporous polymers (CMPs) are a sub-class of porous materials that are related to structures such as zeolites, metal-organic frameworks, and covalent organic frameworks, but are amorphous in nature, rather than crystalline. CMPs are also a sub-class of conjugated polymers and possess many of the same properties such as conductivity, mechanical rigidity, and insolubility. CMPs are created through the linking of building blocks in a π-conjugated fashion and possess 3-D networks. Conjugation extends through the system of CMPs and lends conductive properties to CMPs. Building blocks of CMPs are attractive in that the blocks possess broad diversity in the π units that can be used and allow for tuning and optimization of the skeleton and subsequently the properties of CMPs. Most building blocks have rigid components such as alkynes that cause the microporosity. CMPs have applications in gas storage, heterogeneous catalysis, light emitting, light harvesting, and electric energy storage.
IUPAC Polymer Nomenclature are standardized naming conventions for polymers set by the International Union of Pure and Applied Chemistry (IUPAC) and described in their publication "Compendium of Polymer Terminology and Nomenclature", which is also known as the "Purple Book". Both the IUPAC and Chemical Abstracts Service (CAS) make similar naming recommendations for the naming of polymers.
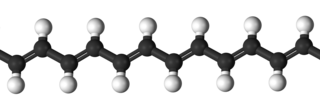
In polymer chemistry, reversible-deactivation radical polymerizations (RDRPs) are members of the class of reversible-deactivation polymerizations which exhibit much of the character of living polymerizations, but cannot be categorized as such as they are not without chain transfer or chain termination reactions. Several different names have been used in literature, which are:

A two-dimensional polymer (2DP) is a sheet-like monomolecular macromolecule consisting of laterally connected repeat units with end groups along all edges. This recent definition of 2DP is based on Hermann Staudinger's polymer concept from the 1920s. According to this, covalent long chain molecules ("Makromoleküle") do exist and are composed of a sequence of linearly connected repeat units and end groups at both termini.
Poly(amidoamine), or PAMAM, is a class of dendrimer which is made of repetitively branched subunits of amide and amine functionality. PAMAM dendrimers, sometimes referred to by the trade name Starburst, have been extensively studied since their synthesis in 1985, and represent the most well-characterized dendrimer family as well as the first to be commercialized. Like other dendrimers, PAMAMs have a sphere-like shape overall, and are typified by an internal molecular architecture consisting of tree-like branching, with each outward 'layer', or generation, containing exponentially more branching points. This branched architecture distinguishes PAMAMs and other dendrimers from traditional polymers, as it allows for low polydispersity and a high level of structural control during synthesis, and gives rise to a large number of surface sites relative to the total molecular volume. Moreover, PAMAM dendrimers exhibit greater biocompatibility than other dendrimer families, perhaps due to the combination of surface amines and interior amide bonds; these bonding motifs are highly reminiscent of innate biological chemistry and endow PAMAM dendrimers with properties similar to that of globular proteins. The relative ease/low cost of synthesis of PAMAM dendrimers (especially relative to similarly-sized biological molecules such as proteins and antibodies), along with their biocompatibility, structural control, and functionalizability, have made PAMAMs viable candidates for application in drug development, biochemistry, and nanotechnology.

In polymer chemistry, graft polymers are segmented copolymers with a linear backbone of one composite and randomly distributed branches of another composite. The picture labeled "graft polymer" shows how grafted chains of species B are covalently bonded to polymer species A. Although the side chains are structurally distinct from the main chain, the individual grafted chains may be homopolymers or copolymers. Graft polymers have been synthesized for many decades and are especially used as impact resistant materials, thermoplastic elastomers, compatibilizers, or emulsifiers for the preparation of stable blends or alloys. One of the better-known examples of a graft polymer is a component used in high impact polystyrene, consisting of a polystyrene backbone with polybutadiene grafted chains.

Tetramethylurea is the organic compound with the formula (Me2N)2CO. It is a substituted urea. This colorless liquid is used as an aprotic-polar solvent, especially for aromatic compounds and is used e. g. for Grignard reagents.

Amalie L. Frischknecht is an American theoretical polymer physicist at Sandia National Laboratories in Albuquerque, New Mexico. She was elected a fellow of the American Physical Society (APS) in 2012 for "her outstanding contributions to the theory of ionomers and nanocomposites including the development and application of density functional theory to polymers". Her research focuses on understanding the structure, phase behavior, and self-assembly of polymer systems, such as complex fluids polymer nanocomposites, lipid bilayer assemblies, and ionomers.
Virgil Percec is a Romanian-American chemist and P. Roy Vagelos Chair and Professor of Chemistry at the University of Pennsylvania. Expert in organic, macromolecular and supramolecular chemistry including self-assembly, biological membrane mimics, complex chiral systems, and catalysis. Pioneered the fields of liquid crystals with complex architecture, supramolecular dendrimers, Janus dendrimers and glycodendrimers, organic Frank-Kasper phases and quasicrystals, supramolecular polymers, helical chirality, Ni-catalyzed cross-coupling and multiple living and self-interrupted polymerizations. Most of these concepts were inspired by Nature and biological principles.
Polyfullerene is a basic polymer of the C60 monomer group, in which fullerene segments are connected via covalent bonds into a polymeric chain without side or bridging groups. They are called intrinsic polymeric fullerenes, or more often all C60 polymers.