
Antimony is a chemical element; it has symbol Sb (from Latin stibium) and atomic number 51. A lustrous grey metal or metalloid, it is found in nature mainly as the sulfide mineral stibnite (Sb2S3). Antimony compounds have been known since ancient times and were powdered for use as medicine and cosmetics, often known by the Arabic name kohl. The earliest known description of the metalloid in the West was written in 1540 by Vannoccio Biringuccio.

In chemistry, an acid–base reaction is a chemical reaction that occurs between an acid and a base. It can be used to determine pH via titration. Several theoretical frameworks provide alternative conceptions of the reaction mechanisms and their application in solving related problems; these are called the acid–base theories, for example, Brønsted–Lowry acid–base theory.
Bioleaching is the extraction or liberation of metals from their ores through the use of living organisms. Bioleaching is one of several applications within biohydrometallurgy and several methods are used to treat ores or concentrates containing copper, zinc, lead, arsenic, antimony, nickel, molybdenum, gold, silver, and cobalt.

In chemistry and manufacturing, electrolysis is a technique that uses direct electric current (DC) to drive an otherwise non-spontaneous chemical reaction. Electrolysis is commercially important as a stage in the separation of elements from naturally occurring sources such as ores using an electrolytic cell. The voltage that is needed for electrolysis to occur is called the decomposition potential. The word "lysis" means to separate or break, so in terms, electrolysis would mean "breakdown via electricity."
Solubility equilibrium is a type of dynamic equilibrium that exists when a chemical compound in the solid state is in chemical equilibrium with a solution of that compound. The solid may dissolve unchanged, with dissociation, or with chemical reaction with another constituent of the solution, such as acid or alkali. Each solubility equilibrium is characterized by a temperature-dependent solubility product which functions like an equilibrium constant. Solubility equilibria are important in pharmaceutical, environmental and many other scenarios.

In chemistry, there are three definitions in common use of the word "base": Arrhenius bases, Brønsted bases, and Lewis bases. All definitions agree that bases are substances that react with acids, as originally proposed by G.-F. Rouelle in the mid-18th century.
In chemistry, an amphoteric compound is a molecule or ion that can react both as an acid and as a base. What exactly this can mean depends on which definitions of acids and bases are being used.

Ethylene oxide is an organic compound with the formula C2H4O. It is a cyclic ether and the simplest epoxide: a three-membered ring consisting of one oxygen atom and two carbon atoms. Ethylene oxide is a colorless and flammable gas with a faintly sweet odor. Because it is a strained ring, ethylene oxide easily participates in a number of addition reactions that result in ring-opening. Ethylene oxide is isomeric with acetaldehyde and with vinyl alcohol. Ethylene oxide is industrially produced by oxidation of ethylene in the presence of a silver catalyst.
An oxyanion, or oxoanion, is an ion with the generic formula A
xOz−
y. Oxyanions are formed by a large majority of the chemical elements. The formulae of simple oxyanions are determined by the octet rule. The corresponding oxyacid of an oxyanion is the compound H
zA
xO
y. The structures of condensed oxyanions can be rationalized in terms of AOn polyhedral units with sharing of corners or edges between polyhedra. The oxyanions adenosine monophosphate (AMP), adenosine diphosphate (ADP) and adenosine triphosphate (ATP) are important in biology.

Silver chloride is an inorganic chemical compound with the chemical formula AgCl. This white crystalline solid is well known for its low solubility in water and its sensitivity to light. Upon illumination or heating, silver chloride converts to silver, which is signaled by grey to black or purplish coloration in some samples. AgCl occurs naturally as the mineral chlorargyrite.
The pedosphere is the outermost layer of the Earth that is composed of soil and subject to soil formation processes. It exists at the interface of the lithosphere, atmosphere, hydrosphere and biosphere. The pedosphere is the skin of the Earth and only develops when there is a dynamic interaction between the atmosphere, biosphere, lithosphere and the hydrosphere. The pedosphere is the foundation of terrestrial life on Earth.

A single-displacement reaction, also known as single replacement reaction or exchange reaction, is an archaic concept in chemistry. It describes the stoichiometry of some chemical reactions in which one element or ligand is replaced by atom or group.
Redox potential is a measure of the tendency of a chemical species to acquire electrons from or lose electrons to an electrode and thereby be reduced or oxidised respectively. Redox potential is expressed in volts (V). Each species has its own intrinsic redox potential; for example, the more positive the reduction potential, the greater the species' affinity for electrons and tendency to be reduced.
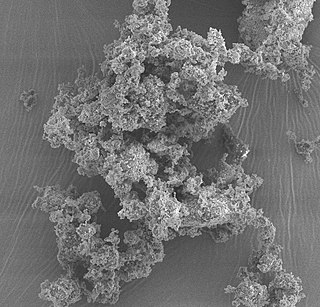
Bioactive glasses are a group of surface reactive glass-ceramic biomaterials and include the original bioactive glass, Bioglass. The biocompatibility and bioactivity of these glasses has led them to be used as implant devices in the human body to repair and replace diseased or damaged bones. Most bioactive glasses are silicate-based glasses that are degradable in body fluids and can act as a vehicle for delivering ions beneficial for healing. Bioactive glass is differentiated from other synthetic bone grafting biomaterials, in that it is the only one with anti-infective and angiogenic properties.
Organoarsenic chemistry is the chemistry of compounds containing a chemical bond between arsenic and carbon. A few organoarsenic compounds, also called "organoarsenicals," are produced industrially with uses as insecticides, herbicides, and fungicides. In general these applications are declining in step with growing concerns about their impact on the environment and human health. The parent compounds are arsane and arsenic acid. Despite their toxicity, organoarsenic biomolecules are well known.
In coordination chemistry, a stability constant is an equilibrium constant for the formation of a complex in solution. It is a measure of the strength of the interaction between the reagents that come together to form the complex. There are two main kinds of complex: compounds formed by the interaction of a metal ion with a ligand and supramolecular complexes, such as host–guest complexes and complexes of anions. The stability constant(s) provide(s) the information required to calculate the concentration(s) of the complex(es) in solution. There are many areas of application in chemistry, biology and medicine.
Organoantimony chemistry is the chemistry of compounds containing a carbon to antimony (Sb) chemical bond. Relevant oxidation states are SbV and SbIII. The toxicity of antimony limits practical application in organic chemistry.
A metal ion in aqueous solution or aqua ion is a cation, dissolved in water, of chemical formula [M(H2O)n]z+. The solvation number, n, determined by a variety of experimental methods is 4 for Li+ and Be2+ and 6 for most elements in periods 3 and 4 of the periodic table. Lanthanide and actinide aqua ions have higher solvation numbers (often 8 to 9), with the highest known being 11 for Ac3+. The strength of the bonds between the metal ion and water molecules in the primary solvation shell increases with the electrical charge, z, on the metal ion and decreases as its ionic radius, r, increases. Aqua ions are subject to hydrolysis. The logarithm of the first hydrolysis constant is proportional to z2/r for most aqua ions.